If you have a project involving a radar system, we can help you with the engineering and design by providing Ansys simulation software, training, and support as well as our consulting services.
The Range-Doppler setup within HFSS SBR+ provides a highly optimized simulation capability for radar systems used in modern automotive radar and aerospace applications. It simulates the response of a Range-Doppler radar system in a complex large-scale environment, such as an automotive radar viewing a traffic scenario or an airborne radar viewing objects on the ground. The results are presented as Range-Doppler colormap images and animations, with the simulation workflow contained entirely within the Ansys Electronics Desktop environment.
The SBR+ radar simulation is massively accelerated relative to conventional implementations using Ansys proprietary Accelerated Doppler Processing (ADP) technology. The ADP technique eliminates the need to separately solve hundreds of individual chirps in microscopic time-stepping of the dynamic scene. ADP also provides improved image quality by eliminating Doppler artifacts of the ray-tracing solution.
Radar modeling capabilities in HFSS SBR+ are continually increasing to enable more advanced and faster simulations. HFSS SBR+ uses a high-frequency ray-tracing method that efficiently computes scattering from electrically large geometries. ADP was originally introduced in 2019 R2 to perform fast radar scene simulations using Pulse-Doppler waveforms. In 2021 R1, the chirp-sequence FMCW waveform was added to model this popular radar signal type.
In 2022 R1, a powerful new capability named Fast Frequency Looping has been added to further reduce the runtime of Range-Doppler simulations. This innovative capability applies to both types of radar waveforms and can decrease simulation times by an additional order of magnitude. Fast frequency looping is applicable for waveforms having up to 10% relative bandwidth. Many radar designs meet this requirement on the signal bandwidth, such as those operating in the 24 GHz and 77 GHz frequency bands. Figure 1 shows an example Range-Doppler simulation of an automotive radar module entering a traffic intersection. The radar operates at 77 GHz and is composed of 8 transmitters and 16 receivers with a total of 128 channels. The scene includes three vehicles, a pedestrian, and a bicyclist moving towards or away from the four-way intersection.
Figure 1:
HFSS SBR+ simulation of 128-channel 77 GHz radar module viewing a traffic intersection
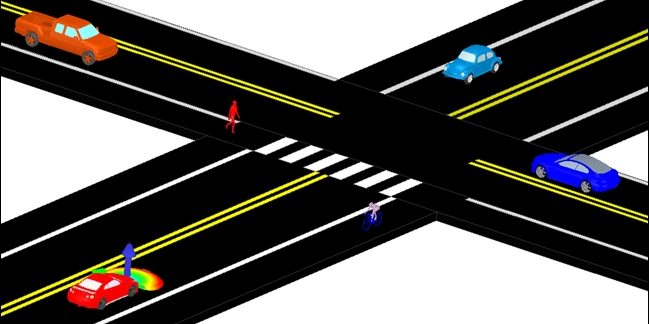
The Range-Doppler solution setup window in HFSS SBR+ is shown in Figure 2. Range-Doppler radar systems operate by issuing a sequence of many pulses or chirps across a coherent processing interval (CPI). For this simulation, the waveform type is specified as Pulse-Doppler with range and velocity performance parameters entered to calculate the associated waveform and CPI parameter values. Note that in this case the radar waveform bandwidth is approximately 1% of the center frequency. The new Fast Frequency Looping feature available in 2022 R1 is enabled by simply selecting the checkbox on the Options tab.
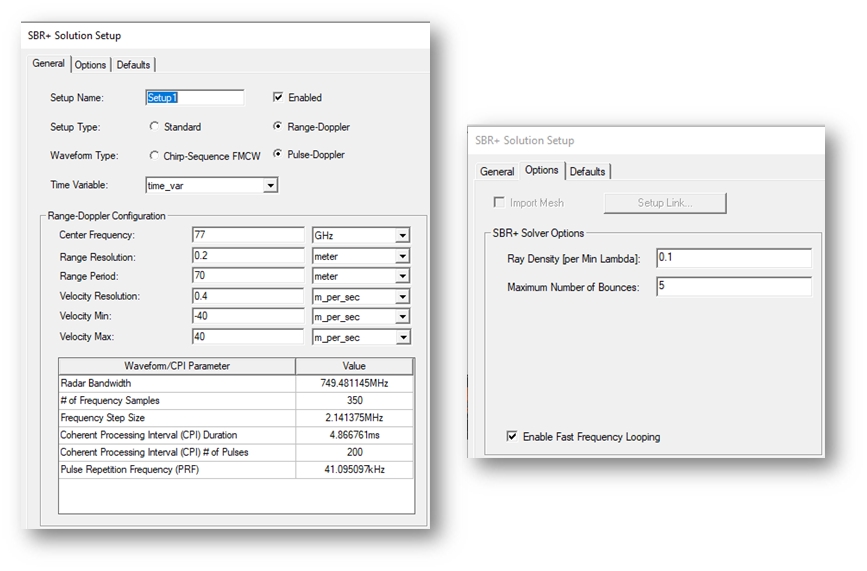
Figure 2:
Solution setup for 77 GHz Range-Doppler simulation with Fast Frequency Looping
The results of radar simulations with and without Fast Frequency Looping enabled are shown in Figure 3. The Fast Frequency Looping results are from HFSS SBR+ 2022 R1, while the results without Fast Frequency Looping are from HFSS SBR+ 2021 R2. No appreciable differences are discernable in the range profiles and Range-Doppler images, confirming that simulation results for the two cases are practically identical.
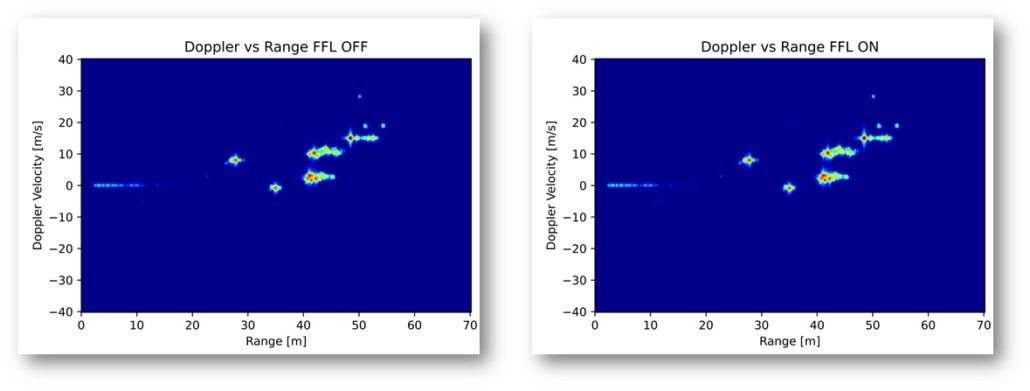
Figure 3:
Range-Doppler simulation results with and without Fast Frequency Looping
Figure 4 shows simulation results displayed as a hemispherical range-angle polar plot. This demonstrates how the multiple-input multiple-output (MIMO) radar can be used to determine direction of arrival. Each target object in the scene is identified at a unique location from the view of the radar module, with the vehicles producing larger reflected signals than the pedestrian and bicyclist.
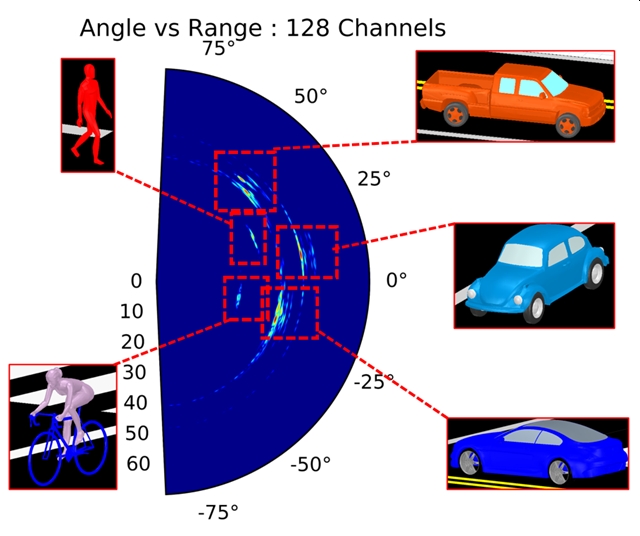
Figure 4:
Simulated Range-Doppler target returns for the scenario in Figure 1
Finally, Figure 5 shows the solution profiles solved on a computer with 6 processor cores. This type of multiple-core configuration is representative of a modern laptop computer. The simulation without Fast Frequency Looping completed in 1 hour 43 minutes and used 159 MB of memory, while the simulation with Fast Frequency Looping completed in only 6 minutes and used a similar 170 MB of memory. In this case, the use of Fast Frequency Looping yielded a 17X faster runtime and produced the same simulation results. Upgrade to 2022 R1 to harness this powerful new capability for your Range-Doppler radar simulations!
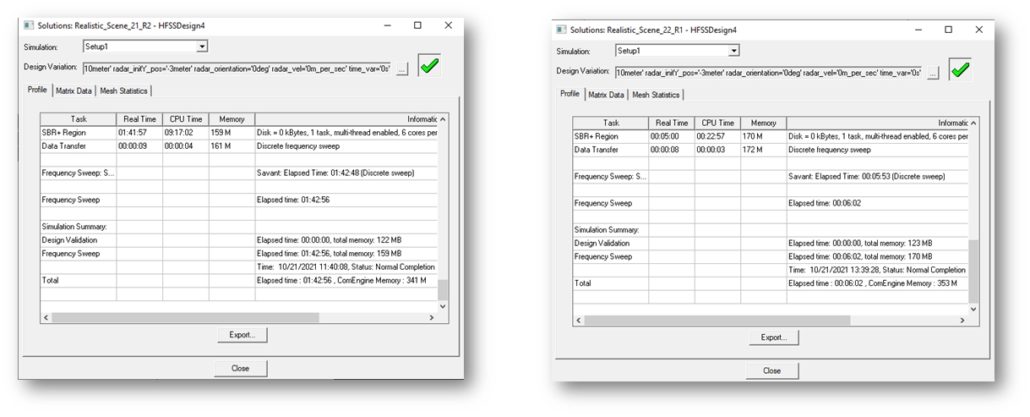
Figure 5:
Range-Doppler solution profiles with and without Fast Frequency Looping